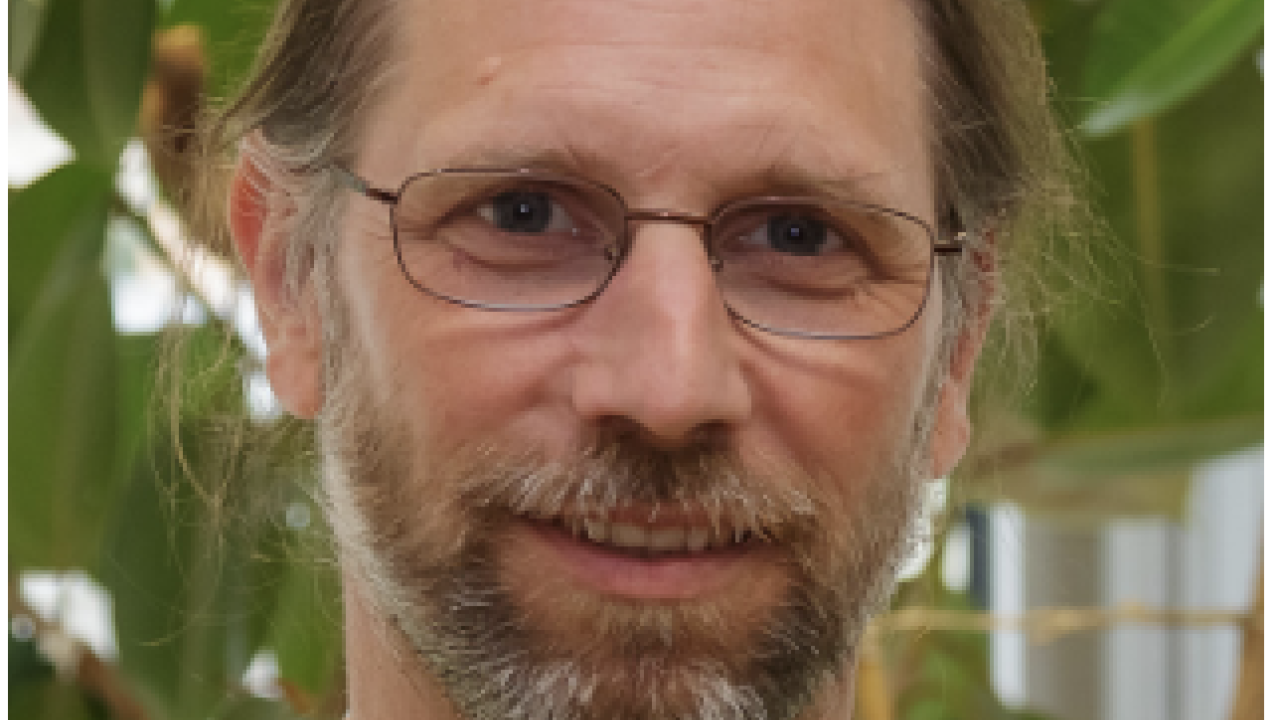
Fundamental Physics to Build Computers of the Future
Quick Summary
- Hartmut Haeffner, a 2022 Fellow with the UC Noyce Initiative, studies the properties and behaviors of isolated atoms— potential building blocks for quantum computers
- This article was originally published by the UC Berkeley Office of Research.
When he was 12 years old, Hartmut Haeffner got his first computer— a Commodore 64. On the C64, Haeffner learned to program, first for fun and then as a job, using the computer to track sporting events in his native Germany. He was fascinated with both what the computer could do, and the underlying technology that made it possible.
Now, as a professor of physics and a 2022 Fellow with the UC Noyce Initiative, Haeffner studies the properties of isolated, charged atoms. His work illuminates how these lone atoms act in unusual ways that could let them become the building blocks of new quantum computers—powerful machines that are a far jump, in both technology and capability, from the C64.
Unlike conventional computers, which store information in binary bits (represented by either a 0 or 1), quantum computers use qubits, which can exist in a state of superposition (representing 0 and 1 simultaneously). This lets the computers solve certain types of problems that conventional computers are not able to tackle.
The atoms that Haeffner studies can take on quantum properties and assume the role of qubits. Understanding the basic rules that govern the atoms’ behavior is vital to both basic science and to pushing quantum computers forward, he says.
“Computing wasn’t originally developed so we could sit at a desktop computer and have a conversation on Zoom,” Haeffner says. “They were developed to solve fundamental questions in science. Similarly, my research is extremely fundamental, and mostly just driven by curiosity. But it’s exciting to also be able to say that in thirty or fifty years, this technology could be revolutionary and change people’s lives in ways we can’t yet imagine.”
In the future, he predicts, quantum computers could become useful mostly in technical fields— for modeling the behavior of molecular systems encoding data in new ways for secure communication, searching large data sets, and optimization problems, for instance.
Beginning with Measurement
After Haeffner spent his teenage years programming, friends and relatives assumed he would study computer science in college. But when he enrolled at the University of Mainz, he chose physics. The field captivated him and he became especially interested in extremely precise measurement techniques that could reveal the behavior of atoms and electrons.
“How incredible is it that there are measurement techniques that let us look at individual atoms and basically be able to say, this atom is in one state, this atom is in another state?” Haeffner says.
In a quantum computer, information can be encoded in these properties of atoms, so being able to measure them is key to developing new computing methods. Haeffner’s graduate research revolved around using such techniques to study electrons inside the extremely strong electric fields created by highly charged ions. The work aimed to answer long-standing questions about fundamental laws of physics.
Then, during a postdoctoral fellowship at the U.S. National Institute for Standards and Technology (NIST), Haeffner discovered that the tools he was already using had potential applications in quantum information sciences. At the time, quantum computing was just picking up steam, but many hypothesized quantum computers processed data using trapped ions— very similarly to the system Haeffner was already working with.
Scientists had a plethora of questions, however, about how these ions could be controlled with laser light, and how long they maintained their properties— a critical measurement since it reflects how long they can store information. Haeffner’s technologies aim to make these kind of trapped ion systems more precise, through better control of the ions and more efficient traps to hold the ions in place.
A Goal to Reduce Error
For the past 25 years— and at Berkeley since 2009— Haeffner has been using precision measurement techniques to study trapped ions. Much of his work is inspired by questions about how quantum systems work in the first place and how to make trapped ions the ideal qubits for eventual quantum computers.
Today’s quantum computers, in various stages of development, are not yet being used commercially, in part because of their high error rates. Qubits are inherently sensitive to changes in their environment; slight fluctuations in their surroundings can change their state—and therefore the data they contain. Another problem with existing quantum computers is that researchers have struggled to make them bigger while maintaining precision control; the very same methods which are applicable to control 10 qubits, will likely not work for 100 qubits, he says. To tackle real-world problems, quantum computers will likely need thousands of highly controllable qubits.
Haeffner’s research could eventually help him overcome these shortcomings. He’s studying the benefits of using trapped electrons, for instance, rather than trapped ions.
“The drawback of ions is that they’re relatively heavy, which makes many operations slow,” he says. “If we replace the ions with electrons, we think the technology might be faster.”
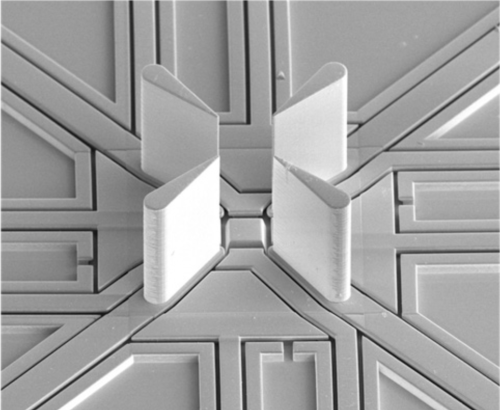
With funding from the UC Noyce Initiative, Haeffner’s group is also constructing new three-dimensional structures to contain trapped ions or electrons— a change that he thinks could reduce error rates. Traditionally, flat chips have been used to generate and organize the electromagnetic fields that coax the ions into their free-floating positions.
“When we first started making ion traps, that made sense, but nowadays there are fabrication methods where you can build three-dimensional structures with high precision at the micron scale,” Haeffner says.
Haeffner’s goals are to build better ion and electron traps and speed up operations and, as a result, reduce error in quantum computing.
“We think that these new technologies can suppress the error rate by about 30, which could really prove crucial in moving forward with feasible quantum computers,” he says.
Haeffner does not like to speculate about the future applications of quantum computing much; he says his role is to stay motivated by basic physics. Quantum laptops— or even quantum computers that can do anything “useful” beyond helping model quantum physics problems— are decades off, he believes.
“It’s a big adventure to see what can be done and what emerges from this research,” he says.
This article was originally published by UC Berkeley Office of Research.